Snow falls differently on the nanoscale
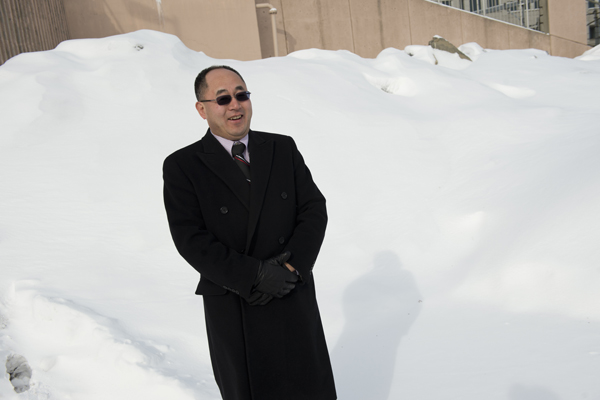
This time of year it’s not hard to imagine the world buried under a smooth blanket of snow. A picnic table on a flat lawn eventually vanishes as trillions of snowflakes collect around it, a crystalline sheet obscuring the normally-visible peaks and valleys of our summertime world.
This is basically how scientists understand the classical theory of crystalline growth. Height steps gradually disappear as atoms of a given material—be it snow or copper or aluminum—collect on a surface and then tumble down to lower heights to fill in the gaps. The only problem with this theory is that it totally falls apart when applied to extremely small situations—i.e., the nanoscale.
Hanchen Huang, professor and chair of Northeastern University’s Department of Mechanical and Industrial Engineering, has spent the last 10 years revising the classical theory of crystal growth that accounts for his observations of nanorod crystals. His work has garnered the continued support of the U.S, Department of Energy’s Basic Energy Science Core Program.
Nanorods are miniscule fibers grown perpendicular to a substrate, each one about 100,000 times thinner than a human hair. Surface steps, or the minor variations in the vertical landscape of that substrate, determine how the rods will grow.
“Even if some surface steps are closer and others more apart at the start, with time the classical theory predicts they become more equalized,” Huang said. “But we found that the classical theory missed a positive feedback mechanism.”
This mechanism, he explained, causes the steps to “cluster,” making it more difficult for atoms to fall from a higher step to a lower one. So, instead of filling in the height gaps of a variable surface, atoms in a nanorod crystal localize to the highest levels.
“The taller region gets taller,” Huang said. “It’s like, if you ever play basketball, you know the taller guys will get more rebounds.” That’s basically what happens with nanorod growth.
Huang’s theory, which was published in the journal Physical Review Letters this year, represents the first time anyone has provided a theoretical framework for understanding nanorod crystal growth. “Lots of money has been spent over the past decades on nanoscience and nanotechnology,” Huang said. “But we can only turn that into real-world applications if we understand the science.”
Indeed, his contribution to understanding the science allowed him and his colleagues to predict the smallest possible size for copper nanorods and then successfully synthesize them. Not only are they the smallest nanorods ever produced, but with Huang’s theory he can confidently say they are the smallest nanorods possible using physical vapor deposition.
The material has major implications for commercial applications, including a kind of metallic glue that can fuse two pieces of metal together at room temperature, in ambient environment, and with very little pressure input. This technology may enable ambient soldering without the need of poisonous lead, and could therefore be extremely valuable to the semiconductor industry, which pervades society through the ubiquitous use of handheld and other computer devices.